Lung Ventilators
Although artificial
ventilation of the lungs is less used in veterinary than medical
anesthesia, it is frequently useful and its use is occasionally
mandatory. The primary indication for artificial ventilation is
pulmonary hypoventilation, which may be caused by central depression,
muscle weakness or paralysis (for example, during the use of neuromuscular
blocking drugs), and thoracic trauma or surgery. It must be
noted that IPPV has profound effects on the distribution of pulmonary
ventilation and blood flow,
and systemic hemodynamics. For example, IPPV results in a significant
reduction in cardiac output, particularly in anesthetised horses,
but further discussion of these effects is beyond the scope of this
article.
The most commonly used method of artificial ventilation
in veterinary anesthesia is Intermittent Positive Pressure Ventilation
(IPPV) which, as its name suggests, consists of the intermittent
application of postive pressure to the airway. (Other forms of lung
ventilation include subatmospheric pressure applied to the chest
wall, as in the 'iron lung', and high frequency oscillation, but
these are little used in veterinary practice.)
Although IPPV may easily and effectively be performed
by manually squeezing the breathing bag and occluding the pop-off
valve or other gas outlet, this is inconvenient for long periods
and is physically exhausting when used in large animals such as
horses and cattle. Many mechanical devices have been developed that
aid the anesthetist by performing this function automatically.
Types of
Ventilator
The classification
of ventilators is somewhat complicated, and this is exacerbated
by the fact that the same ventilator may function differently depending
on how the controls are set. However, two of the most commonly used
types of ventilator used in veterinary anesthesia are bag-squeezers
and minute volume dividers. Ventilators designed for long-term ventilation
in intensive care units are not usually suitable for use in anesthesia.
Bag Squeezers
As their name
suggests, bag-squeezers replace the breathing bag, and usually also
the pop-off valve, in the breathing circuit. Although some have
been designed that compress the bag or bellows mechanically, using,
for example, an eccentric cam or crank arrangement, most are of
the bag-in-bottle type. Here, the breathing bag is enclosed in a
rigid container and is squeezed by gas pressure applied to the inside
of the container. The original bag has now usually been replaced
by a concertina-type bellows.
Operation of
a typical bag-squeezer (below) is as follows. During inspiration,
the inspiratory valve (V1) is opened, allowing gas from the low-pressure
system to enter the container and squeeze the bellows. The expiratory
valves (V2 and V3) are closed, so gas from the bellows is directed,
via the breathing circuit, into the lungs. During expiration, the
inspiratory valve (V1) closes, the expiratory valve (V2) opens and
gas flows from the patient circuit into the bellows. Once the bellows
is full, the pop-off valve (V3) opens and any excess gas is vented
from the circuit.
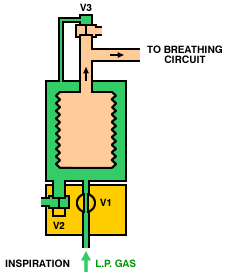
Many older ventilators
of this type are of the descending bellows design, in which the
bellows hangs from the top of the assembly (as above). The problem
with this arrangement is that if the ventilator becomes disconnected
or the circuit develops a leak, the ventilator will continue to
cycle as normal (since, during inspiration, the bellows will be
squeezed and empty as usual and, during expiration, the bellows
will expand under its own weight and fill with gas from the atmosphere).
More
modern ventilators are of the ascending bellows type. Here, exhalation
from the patient during expiration causes the bellows to expand
upwards. If a leak or disconnection occurs, the bellows will not
fill during the expiratory phase since there is nothing to make
it expand. This arrangement is markedly safer than the older descending
bellows design since it will be immediately obvious if disconnection
has occurred.
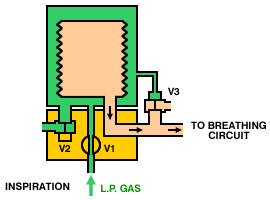
Bag squeezers
are used by substituting them for the breathing bag in circle absorber
systems (with the pop-off valve on the circle closed) and Mapleson
D systems (Modified Bain or T-Piece). They cannot be used in Mapleson
A systems (Magill or Lack) owing to the different position of the
pop-off valve in these circuits.
It will be noted that the minute volume of ventilation
(as determined by the ventilator) is completely independent of the
fresh gas flow rate delivered to the breathing circuit.
The controller unit, which determines the pattern
of ventilation, may be mechanical, fluidic or, more recently, electronic.
Minute Volume
Dividers
In contrast
with bag-squeezers, which simply replace the breathing bag on an
existing circuit, minute volume dividers are self-contained circuits
which take the fresh gas flow rate, divide it up and deliver it
to the patient in individual breaths. It follows, again in contrast
with bag-squeezers, that the minute volume of ventilation is equal
to the fresh gas flow rate.
The operation of a typical conventional minute
volume divider is illustrated below. Fresh gas from the common gas
outlet of the anesthesia machine flows continuously into the circuit.
During inspiration, the inspiratory valve (V1) opens, the expiratory
valve (V2) closes, the weighted or spring-loaded bellows empties
and the lungs are inflated. During expiration, the inspiratory valve
closes, the expiratory valve opens, and the patient exhales, expired
gas being vented from the system.
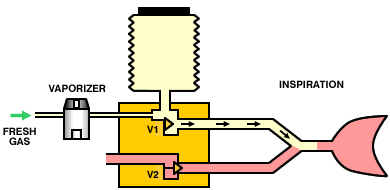
A variation
on this theme is to replace the entire anesthesia machine with a
microprocessor-controlled minute volume divider. The machine is
connected directly to a low-pressure oxygen supply (there are no
external flowmeters). During inspiration, the inspiratory valve
(V1) opens, the expiratory valve (V2) closes, and gas flows through
the vaporizer into the lungs. During expiration, the inspiratory
valve closes, the expiratory valve opens and the patient exhales.
The operation of the valves is controlled by a microprocessor which
can calculate the required tidal volume and respiratory rate (and,
therefore, minute volume) from the patient's body weight. By partially
opening the inspiratory valve and opening the expiratory valve,
the system can also operate as a Mapleson E circuit.
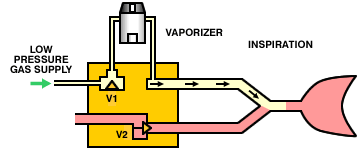
This provides
a compact and convenient ventilator for small animal use. The very
high flow through the vaporizer during inspiration (up to 60 l/min)
may lead to inaccurate output and leaks.
Controls
Every ventilator
needs a means of controlling tidal volume and respiratory rate.
However, the method by which these are controlled varies widely
between makes and models of ventilator. For example, tidal volume
may be controlled:
- Directly,
by adjustment of the excursion of the bellows.
- By adjustment
of the peak inspiratory pressure.
- By adjustment
of the inspiratory time.
- More than
one of the above.
Similarly, respiratory
rate may be controlled or influenced:
- Directly,
by a respiratory frequency control.
- By adjustment
of the inspiratory and/or expiratory time.
- By the tidal
volume or peak inspiratory pressure.
- By the inspiratory
and expiratory flow rate.
- By the fresh
gas flow rate (in the case of minute volume dividers).
Settings that
may be adjusted on different ventilators may include:
- Tidal volume
- Peak inspiratory
pressure
- Respiratory
rate
- Inspiratory/expiratory
time ratio
- Inspiratory
time
- Expiratory
time
- Inspiratory
flow rate
- Expiratory
flow rate
- Inspiratory
hold
- Inspiratory
trigger effort
- Positive
end expiratory pressure (PEEP)
In view of the
large variety of controls on different ventilators, it is impossible
in the space available to give specific instructions on the use
of every specific make and model of ventilator: users should become
familiar with the operation of the ventilators that they have in
their facility. However, some general guidelines can be offered:
- Look at the
patient to make sure that the respiratory pattern looks reasonable.
- A good starting
point for tidal volume is around 10 ml/kg body weight, although
this may need to be adjusted.
- The peak
inspiratory pressure should be kept as low as possible in order
to avoid pulmonary barotrauma and adverse hemodynamic effects.
Peak pressure should generally not exceed 1.5 kPa (15 cmH2O)
in small animals (dogs and cats), which have highly compliant
chest walls. Higher peak inspiratory pressure (3 kPa, 30 cmH2O
or more) will usually be required in large animals (horses and
cattle). If the chest wall is opened, lower pressures will be
necessary in all species (since the stiffness of the chest wall
has been removed).
- Respiratory
rate of 10 - 15 breaths per minute in small animals and 8 - 10
breaths per minute in large animals.
- The optimal
inspiratory to expiratory time ratio reflects a compromise between
the beneficial effects of a longer inspiratory time on the distribution
of ventilation within the lung and the adverse hemodynamic effects
of prolonged positive intrathoracic pressure. An inspiratory:expiratory
time ratio of between 1:2 and 1:3 is usually satisfactory.
- The definitive
criteria for adequacy of pulmonary ventilation are arterial blood
gas analysis and capnography (measurement of the end-tidal CO2
concentration). Blood gas analysis is still rather expensive for
general practice, but capnography is becoming increasingly affordable.
Patient
Connector 
|